4G LTE Air Interface
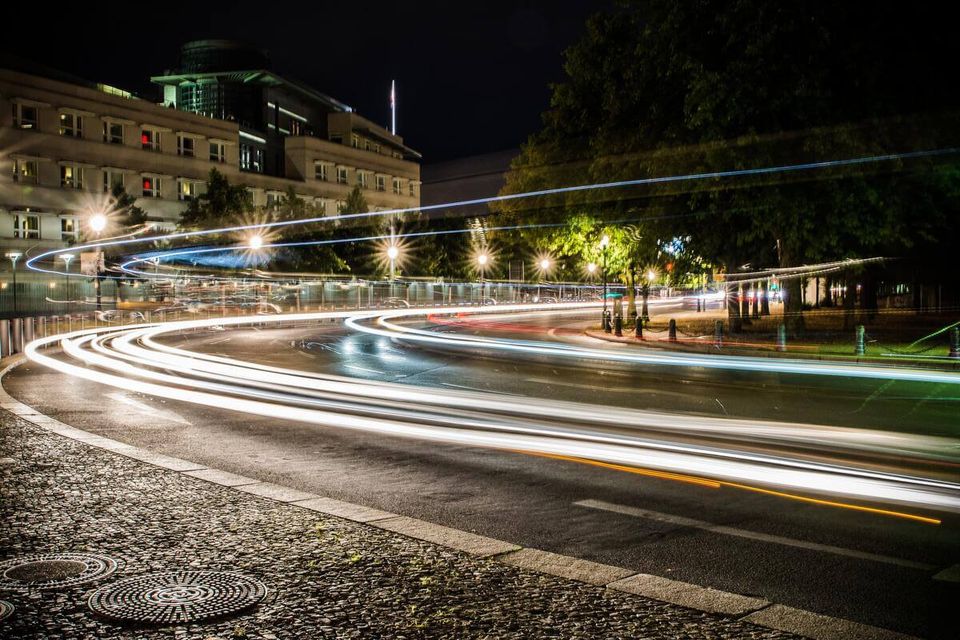
Introduction:
Long-Term Evolution (LTE) is a wireless broadband technology that provides high-speed data services to mobile devices. The LTE air interface is the radio frequency (RF) part of the LTE system. It is the interface between the LTE base station (eNodeB) and the mobile device (UE), and it defines how data is transmitted wirelessly between the two devices. In this article, we will discuss the LTE air interface in detail, including its technical aspects, protocols, and standards.
Evolution of the Air Interface:
The LTE air interface is the result of continuous development in wireless communication technologies. It has evolved from the first generation (1G) analog cellular systems to the fourth generation (4G) LTE technology. The evolution of the air interface has been driven by the need for higher data rates, better spectral efficiency, and more efficient use of the radio spectrum.
The first-generation cellular systems used analog modulation techniques such as frequency modulation (FM) and amplitude modulation (AM) to transmit voice signals. The second-generation (2G) systems introduced digital modulation techniques such as time division multiple access (TDMA) and code division multiple access (CDMA) to improve spectral efficiency and allow for the transmission of data. The third-generation (3G) systems introduced wideband CDMA (WCDMA) and high-speed packet access (HSPA) to increase data rates and support advanced services such as mobile video.
The LTE air interface is based on the Orthogonal Frequency Division Multiplexing (OFDM) modulation scheme, which provides high data rates and excellent spectral efficiency. It also includes advanced features such as multiple antenna technologies (MIMO) and adaptive modulation and coding (AMC) to further improve data rates and spectral efficiency.
Architecture of the LTE Air Interface:
The LTE air interface consists of three main components: the physical layer, the medium access control (MAC) layer, and the radio resource control (RRC) layer. Each layer performs specific functions to ensure the efficient transmission of data over the air interface.
Physical Layer:
The physical layer is responsible for the transmission and reception of data over the air interface. It defines the modulation and coding schemes used for transmission, as well as the frequency and time domain characteristics of the signals. The physical layer also includes the MIMO and AMC features to improve data rates and spectral efficiency.
The physical layer uses OFDM modulation, which divides the available spectrum into multiple subcarriers that are orthogonal to each other. Each subcarrier is modulated with a data symbol, and the resulting signal is transmitted over the air interface. The OFDM modulation scheme provides high data rates and excellent spectral efficiency, as it allows for the transmission of multiple subcarriers simultaneously.
The physical layer also includes the MIMO feature, which uses multiple antennas at the transmitter and receiver to increase data rates and spectral efficiency. MIMO technology takes advantage of the spatial diversity of the wireless channel to transmit multiple data streams simultaneously over the same frequency band.
The AMC feature is used to adapt the modulation and coding schemes used for transmission to the channel conditions. The AMC algorithm selects the most appropriate modulation and coding scheme based on the channel quality, which varies over time and location. This ensures that the highest possible data rates are achieved while maintaining reliable transmission.
Medium Access Control Layer:
The MAC layer is responsible for managing the access to the radio channel and coordinating the transmission and reception of data between the UE and the eNodeB. The MAC layer uses a hybrid automatic repeat request (HARQ) protocol to ensure reliable transmission over the air interface.
The HARQ protocol is a combination of error correction and retransmission techniques. When a data packet is transmitted over the air interface, the receiver sends an acknowledgement (ACK) or negative acknowledgement (NACK) message to the sender. If the message is an ACK, the transmission is considered successful, and the next packet can be sent. If the message is a NACK, the sender retransmits the packet until it is successfully received.
The MAC layer also includes the scheduling function, which determines which UE is allowed to transmit data and when. The scheduling function uses a prioritization scheme based on the quality of service (QoS) requirements of different applications and the channel conditions.
Radio Resource Control Layer:
The RRC layer is responsible for the establishment, maintenance, and release of the radio connections between the UE and the eNodeB. It also manages the configuration of the radio resources, including the frequency bands, modulation and coding schemes, and channel quality indicators (CQIs).
The RRC layer uses a state machine to manage the different states of the radio connection. The initial state is the idle state, in which the UE is not connected to the network. When the UE wants to establish a connection, it sends a connection request message to the eNodeB. If the request is accepted, the RRC layer moves to the connected state, and data can be transmitted over the air interface.
Protocols Used in the LTE Air Interface:
The LTE air interface uses several protocols to ensure the efficient transmission of data over the air interface. These protocols include:
Radio Resource Control (RRC) Protocol:
The RRC protocol is used to establish, maintain, and release the radio connections between the UE and the eNodeB. It also manages the configuration of the radio resources, including the frequency bands, modulation and coding schemes, and CQIs.
Packet Data Convergence Protocol (PDCP):
The PDCP protocol is responsible for the compression and encryption of the data packets transmitted over the air interface. It also provides header compression to reduce the overhead of the data packets.
Radio Link Control (RLC) Protocol:
The RLC protocol is responsible for the segmentation and reassembly of the data packets transmitted over the air interface. It also provides error correction and retransmission capabilities through the HARQ protocol.
Medium Access Control (MAC) Protocol:
The MAC protocol is responsible for managing the access to the radio channel and coordinating the transmission and reception of data between the UE and the eNodeB. It also includes the scheduling function to determine which UE is allowed to transmit data and when.
Physical Layer (PHY) Protocol:
The PHY protocol is responsible for the transmission and reception of data over the air interface. It defines the modulation and coding schemes used for transmission, as well as the frequency and time domain characteristics of the signals. It also includes the MIMO and AMC features to improve data rates and spectral efficiency.
Standards Used in the LTE Air Interface:
The LTE air interface is governed by several standards developed by the 3rd Generation Partnership Project (3GPP). These standards define the technical specifications and requirements for the LTE air interface, ensuring interoperability between different vendors and networks.
The LTE air interface standards include:
LTE Release 8: Released in 2008, LTE Release 8 was the first commercial release of the LTE standard. It included several key features such as OFDM modulation, MIMO, and AMC, and provided data rates of up to 100 Mbps.
LTE Release 9: Released in 2009, LTE Release 9 included several enhancements to the LTE standard, including the support for small cell deployments, improved handover performance, and the introduction of dual-carrier HSPA.
LTE Release 10: Released in 2011, LTE Release 10 introduced several new features to the LTE standard, including the support for carrier aggregation, which allows for the aggregation of multiple carriers to increase data rates, and the introduction of relay nodes, which enable the extension of coverage in areas with poor signal quality.
LTE Release 11: Released in 2012, LTE Release 11 included several enhancements to the LTE standard, including the support for Coordinated Multipoint (CoMP), which improves the data rates and coverage by coordinating the transmission and reception of data between multiple eNodeBs.
LTE Release 12: Released in 2014, LTE Release 12 included several new features to the LTE standard, including the support for LTE Broadcast, which enables the transmission of multicast and broadcast services over the LTE network, and the introduction of Device-to-Device (D2D) communication, which enables direct communication between UEs without going through the network.
LTE Release 13: Released in 2016, LTE Release 13 included several enhancements to the LTE standard, including the support for narrowband IoT (NB-IoT), which is a low-power, low-bandwidth technology designed for the Internet of Things (IoT) applications.
LTE Release 14: Released in 2017, LTE Release 14 included several new features to the LTE standard, including the support for License Assisted Access (LAA), which enables the use of unlicensed spectrum in combination with licensed spectrum to increase data rates, and the introduction of LTE-M, which is a low-power, low-bandwidth technology designed for the IoT applications.
LTE Release 15: Released in 2018, LTE Release 15 included several enhancements to the LTE standard, including the support for massive Machine-Type Communications (mMTC), which is designed for the massive deployment of low-power IoT devices, and the introduction of enhanced Carrier Aggregation (eCA), which enables the aggregation of up to 32 carriers to increase data rates.
Conclusion:
The LTE air interface is a critical component of the LTE network, enabling the transmission of data between the UE and the eNodeB over the radio channel. The LTE air interface uses advanced technologies such as OFDM modulation, MIMO, and AMC to achieve high data rates and spectral efficiency. The LTE air interface is governed by several standards developed by the 3GPP, which define the technical specifications and requirements for the LTE air interface, ensuring interoperability between different vendors and networks. The LTE air interface is a constantly evolving technology, with new features and enhancements being introduced in each new release of the LTE standard, to improve performance, increase data rates, and support new applications and services.