eNB Architecture Evolution
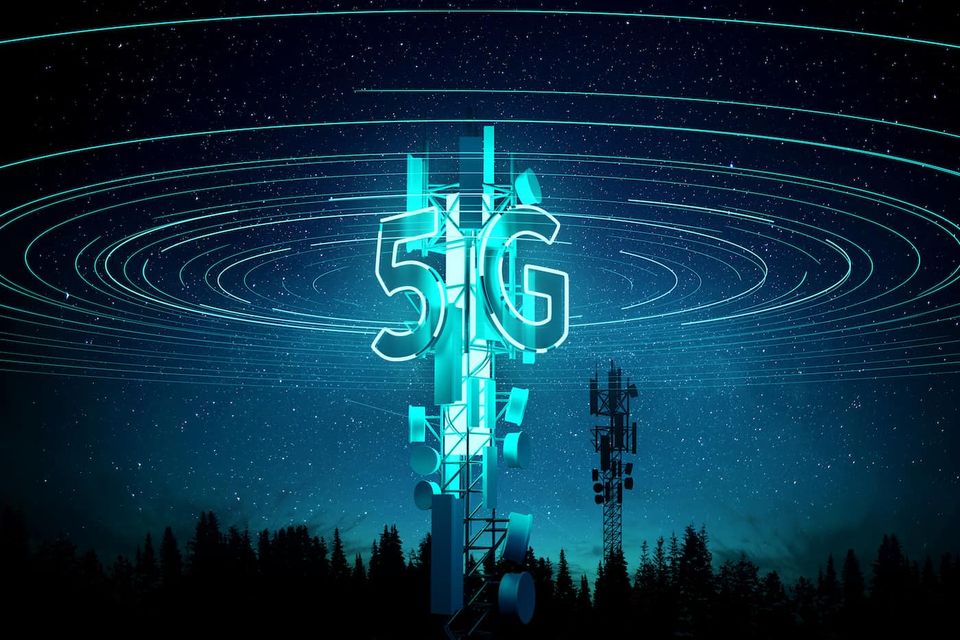
The evolution of eNB (evolved NodeB) architecture has been driven by the need for faster data rates, improved spectral efficiency, and the support of new services and applications. This evolution has resulted in the introduction of new functionalities and interfaces in the eNB architecture. In this article, we will discuss the technical details of the eNB architecture evolution.
eNB Architecture Overview
The eNB is a key element in the LTE (Long-Term Evolution) network. It is responsible for controlling the radio access network (RAN) and connecting to the core network (CN). The eNB is a network element that has undergone significant changes in architecture over the years.
Initially, the eNB architecture consisted of a single functional entity that included both the control plane and user plane functions. This architecture was known as an all-in-one eNB. The all-in-one eNB architecture was not scalable, as it did not allow for the addition of new functionalities or interfaces.
To address these limitations, the eNB architecture evolved into a split architecture, which separated the control plane and user plane functions into two separate entities. The split architecture enabled the addition of new functionalities and interfaces, and improved the scalability of the eNB.
eNB Architecture Evolution
The eNB architecture has evolved through several phases to support the increasing demands of LTE networks. These phases include:
- Release 8: In Release 8, the eNB architecture was an all-in-one entity that included both the control plane and user plane functions. The all-in-one eNB was responsible for radio resource management, radio bearers control, and the delivery of data packets.
- Release 9: In Release 9, the eNB architecture evolved into a split architecture. The control plane and user plane functions were separated into two functional entities, the eNB and the S-GW (serving gateway). The eNB was responsible for radio resource management and radio bearers control, while the S-GW was responsible for the delivery of data packets.
- Release 10: In Release 10, the eNB architecture was further enhanced to support heterogeneous networks (HetNets) and the deployment of small cells. The eNB architecture included new interfaces, such as the X2 interface, which enabled communication between neighboring eNBs and improved the coordination of radio resources. The eNB architecture also included new functionalities, such as the mobility load balancing (MLB) function, which improved the management of user mobility and load balancing between cells.
- Release 11: In Release 11, the eNB architecture was enhanced to support Carrier Aggregation (CA), which enabled the aggregation of multiple carriers to increase the data rate. The eNB architecture included new interfaces, such as the S1-U interface, which enabled the connection between the eNB and the P-GW (packet gateway).
- Release 12: In Release 12, the eNB architecture was further enhanced to support the Internet of Things (IoT) and machine-to-machine (M2M) communications. The eNB architecture included new functionalities, such as the support of low-power devices, improved battery life, and the support of new radio access technologies.
- Release 13: In Release 13, the eNB architecture was enhanced to support enhanced Carrier Aggregation (eCA), which enabled the aggregation of up to 32 carriers to increase the data rate. The eNB architecture also included new functionalities, such as the support of device-to-device (D2D) communications, which enabled direct communication between devices without going through the core network.
- Release 14: In Release 14, the eNB architecture was enhanced to support the LTE Broadcast (eMBMS) service, which enabled the distribution of multimedia content to multiple users simultaneously. The eNB architecture included new functionalities, such as the support of multicast and broadcast services, and the MBMS logical architecture.
- Release 15: In Release 15, the eNB architecture was further enhanced to support 5G New Radio (NR) and the evolution towards a 5G network. The eNB architecture included new interfaces, such as the NG interface, which enabled communication between the eNB and the 5G core network (5GC). The eNB architecture also included new functionalities, such as the support of dual connectivity, which enabled simultaneous connection to both LTE and 5G NR networks.
eNB Architecture Components
The eNB architecture consists of several components that work together to provide radio access to the LTE network. These components include:
- Baseband Unit (BBU): The BBU is responsible for processing the radio signals received from the antennas and generating the radio signals to be transmitted to the user equipment (UE). The BBU also performs radio resource management, such as allocation of radio resources to the UE.
- Remote Radio Head (RRH): The RRH is responsible for transmitting and receiving the radio signals between the UE and the eNB. The RRH is typically located close to the antennas and connected to the BBU via a digital interface.
- Antennas: The antennas are responsible for transmitting and receiving the radio signals between the UE and the eNB. The antennas can be configured in different ways, such as in a single-antenna or multiple-antenna configuration.
- Control Plane: The control plane is responsible for managing the signaling and control information between the UE and the eNB. The control plane includes the RRC (Radio Resource Control) function, which manages the establishment, maintenance, and release of the radio connections between the UE and the eNB.
- User Plane: The user plane is responsible for transmitting the data packets between the UE and the core network. The user plane includes the PDCP (Packet Data Convergence Protocol) function, which compresses and decompresses the data packets, and the RLC (Radio Link Control) function, which provides reliable transmission of the data packets.
eNB Architecture Interfaces
The eNB architecture includes several interfaces that enable communication between the different components of the eNB and between the eNB and the core network. These interfaces include:
- X2 interface: The X2 interface is a high-speed interface that enables communication between neighboring eNBs. The X2 interface enables the coordination of radio resources between neighboring eNBs and improves the handover performance between cells.
- S1 interface: The S1 interface is a high-speed interface that enables communication between the eNB and the core network. The S1 interface includes two sub-interfaces, S1-MME (Mobility Management Entity) and S1-U (User Plane). The S1-MME sub-interface is responsible for managing the signaling and control information between the eNB and the MME (Mobility Management Entity) in the core network. The S1-U sub-interface is responsible for transmitting the data packets between the eNB and the P-GW in the core network.
- NG interface: The NG interface is a high-speed interface that enables communication between the eNB and the 5G core network. The NG interface includes several sub-interfaces, such as the N1 interface, which is responsible for managing the signaling and control information between the eNB and the 5GC.
eNB Architecture Functionalities
The eNB architecture includes several functionalities that enable the management and optimization of the radio access network. These functionalities include:
- Radio Resource Management (RRM): RRM is responsible for managing the radio resources in the eNB, such as allocation of radio resources to the UE and optimization of the radio resources to ensure efficient use of the spectrum.
- Mobility Management (MM): MM is responsible for managing the mobility of the UE within the network, such as handovers between cells and tracking of the UE's location.
- Quality of Service (QoS) Management: QoS management is responsible for ensuring that the UE receives the appropriate level of service based on its requirements and the network's capabilities.
- Security Management: Security management is responsible for ensuring the security of the communication between the UE and the network, such as encryption and authentication.
- Multi-antenna Techniques: Multi-antenna techniques are used to improve the radio performance and increase the capacity of the network. These techniques include MIMO (Multiple Input Multiple Output), beamforming, and diversity.
- Multicast and Broadcast Services: The eNB architecture includes support for multicast and broadcast services, such as MBMS (Multimedia Broadcast Multicast Service).
- Dual Connectivity: Dual connectivity enables simultaneous connection to both LTE and 5G NR networks, providing improved performance and seamless transition between the two networks.
Conclusion
The eNB architecture has evolved over the years to support new functionalities, improve performance, and enable the transition to a 5G network. The eNB architecture includes several components, interfaces, and functionalities that work together to provide radio access to the LTE network. The eNB architecture will continue to evolve to support new technologies and services and enable the transition to a fully integrated 5G network.